TCSPC
Overview
Time-correlated single photon counting (TCSPC) is a powerful method to accurately measure the fluorescence lifetime of samples.
We use a TCSPC setup from Picoquant. Using a laser pulsed at 40 MHz (PDL-800-B), the light intensity is reduced to the level where the photomultiplier detects a photon only after 1% of laser pulses (i.e., count rate <0.4 MHz). Under this condition, fluorescence lifetimes can be calculated from the recorded histograms (i.e., the distribution of time intervals between the laser pulse and the detected photon).
Experimental setup
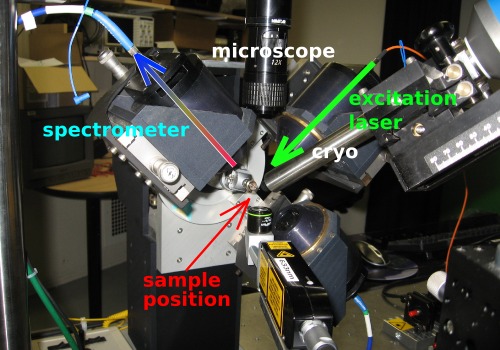
Two perpendicular objectives are used for focussing the excitation laser onto the sample and collecting the emitted light, respectively.
The arrangement of objectives around the sample position is the same for TCSPC as for fluorescence emission measurements, except that the emitted photons are not recorded by a spectrometer but by a photomultiplier. Interferential filters are used in front of the photomultiplier to block the Rayleigh-scattered laser photons and to select specific fluorescence bands.
Specifications of the PDL-800-B pulsed laser diode
- wavelength: 440 nm
- optical power: 1.14 mW at 40 MHz
- pulse duration: <300 ps at 1 mW and <90 ps at 0.3 mW
The setup is described in more detail in a paper by Royant et al. [J. Appl. Cryst. 40, 1105-1112 (2007)].
Software
We use the TimeHarp software by Picoquant for TCSPC experiments.
Example
This TCSPC setup was used for experiments described in a paper by Barros et al. [EMBO J. 28, 298-306 (2009)], as outlined below.

TCSPC histograms of the fluorescence decays of LHC-II at 100 K in dilute solution (blue), single crystals (red), and aggregates (black), for the 680-nm emission band with corresponding fits
(from Barros et al., EMBO J. 28, 298-306 (2009), Fig. 6).
Single crystals of the light harvesting complex of photosystem II (LHC-II) at 100 K show two main emitting species at 680 and 700 nm with average fluorescence lifetimes of 1.31 and 2.63 ns, respectively. Under the same conditions, the fluorescence lifetime of LHC-II aggregates was only 0.39 ns for the 680-nm emission band.
In crystalline LHC-II, the 680-nm lifetime is much longer than expected for a quenched state, but it is consistent with literature values for LHC-II aggregates. Thus it was concluded that the 680 nm lifetime observed in single 3D crystals is inconsistent with a quenched state, which indicates that the crystal structure of LHC-II is the one of the energy-transmitting, unquenched state.
These differences of the quenching behaviour can be understood in the following manner:

(Click for larger image.)
Schematic drawings representing LHC-II in three different phases
(from Barros et al., EMBO J. 28, 298-306 (2009), Fig. 8).
In solution (A), LHC-II trimers are functionally independent and re-emit absorbed light as fluorescence. LHC-II crystals (B) show similar fluorescence. Tight interaction of LHC-II trimers with one another at, e.g., grain boundaries can generate quenching centers, which affects the fluorescence lifetimes. In LHC-II aggregates (C), a high density of quenching centers results in strongly reduced lifetimes.